Cosmology and Structure formation in the Universe
Cosmology is the science that studies the Universe as a
physical entity and explores its overall properties, formation and
evolution. During the very early stages of its evolution the Universe
went through a phase of very rapid expansion (inflationary phase),
which has, among other things, created the initial fluctuations in the
cosmological density field. These fluctuations have grown to generate
the enormous amount of complexity and diversity we currently see
around us. My main interest in cosmology centres on the subfield that
investigates the formation and evolution of the Large Scale Structure
of the Universe. This is one of the most fundamental topics in modern
cosmology.
In order to understand the origin and evolution of the density
fluctuations in the Universe, one has to address a wide range of
topics. In my research I address the following fundamental questions:
1- What is the nature of the initial conditions from which structure
has evolved? 2- What are the physical processes that govern the
evolution of structrue at each stage? 3- What is the connection of
structure formation to the background cosmology and how this
connection could be used? 4- What is the nature and amount of the dark
matter and the dark energy − which are the dominant components of the
energy-density − in the Universe? 5- When and how the baryonis component
(normal matter) have started playing a prominant role in structure
formation in the Universe?
Due to the tremendous improvement in the theory, data analysis
techniques and the amount and diversity of the accumulated
information, a serious examination of these issues has become
feasible. In what follows I list − with some detail − the
main research projects I have been involved with.
- The Epoch of Reionisation of
the Universe
(click here to download a semi-popluar article
I wrote for European Physics News (2009))
The Epoch of Reionisation (EoR) is a term used to describe the period
during which the gas in the Universe went from being almost completely
neutral to a state in which it became almost completely ionised. This
watershed event - which has occurred when the Universe was a few
hundred million years old (about a twentieth of its current age) and
the first radiating objects formed - is intimately linked to many
fundamental questions in cosmology and structure formation and
evolution. Without a clear understanding of the EoR, we will not fully
apprehend how the Universe evolved from its primordial condition to
form the astrophysical object we routinely observe today.
Despite its pivotal role, the EoR is one of the least understood
epochs in the Universe's evolution. A large amount of theoretical
effort, guided by very limited observational evidence, is currently
dedicated to understanding the physical processes that trigger this
epoch, govern its evolution, and what ramifications it had on
subsequent structure formation. In the near future, the LOFAR
telescope, which has the EoR as one of its key projects, is set to
measure the neutral gas fraction in the Universe as a function of
redshift and angular position through the hydrogen hypefine spin-flip
21 cm line. The 21 cm line is, probably, the only obervable tracers of
the gas during the EoR. It allows detailed mapping the EoR as it
progresses in time and space.
|
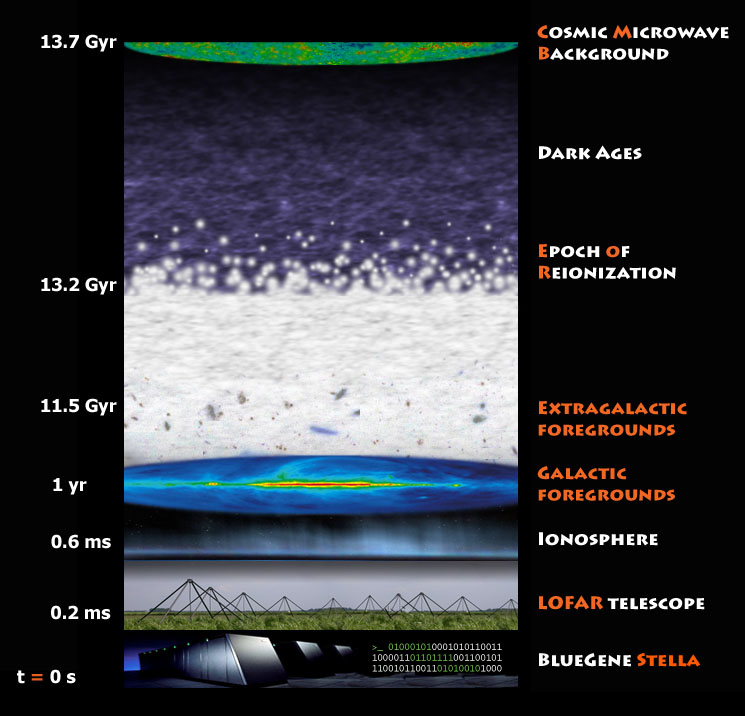 Cartoon of the likely
development of the EoR. About 500,000 years after the Big Bang (z ~
1000) hydrogen recombined and remained neutral for a few hundred
million years (the dark ages). At a redshift, z ~ 10, the first
stars galaxies and quasars began to form, heating and reionising the
hydrogen gas. The neutral intergalactic medium (IGM) can be observed with LOFAR through its
redshifted 21cm spin-flip transition up to redshift 11.5. However,
many atmospheric, galactic and extra-galactic contaminants corrupt the
21 cm signal. | |
Currently, my main objective is to develop the necessary data-analysis
and theoretical tools to, initially, prepare the ground and,
subsequently, fully exploit the LOFAR-EoR data set, expected to be
available within 3-4 years. The synergy between three independent
areas of expetise: theory, observation and data analysis, will
facilitate addressing the fundamental EoR related questions.
- The Nature of the Perturbation
Field: The Universe's PDF.
Determining the nature of the primordial fluctuation field is one the
main goals of the study of Large Scale Structure. Its importance stems
from its sensitivity to the prevailing physical processes in the very
early Universe and its direct relation to the `linear' mass-density
field. Unfortunately, however, recovery of the full probability
distribution function from current data sets has proven
difficult. Therefore two complementary approaches has been
adopted. The first is to assume, as predicted by simple models of
Cosmological-Inflation, that the probability distribution function of
the primordial fluctuations is Gaussian and to measure its moments in
real or Fourier space, especially the power spectrum. The second is to
devise theoretical tests to challenge the Gaussian random field
assumption.
- On the Non-Gaussianity of the CMB:
bispectrum analysis
The CMB presents a very important probe of the background cosmology
and of the distribution of dark and gaseous matter. In particular the
imprint of physical and statistical characteristics of the fluctuation
field on the last scattering surface provides a very direct and clean
test to the Gaussianity of the primordial fluctuations field, an issue
of great importance. Recently, three groups , independently and
relying on different statistical estimates (reduced bispectrum,
skewness in wavelet expansion coefficients and Minkowski functionals
statistics), have reported detections of a non-Gaussian signal in the
publicly available full sky CMB maps obtained by the DMR instrument
aboard the COBE satellite. Together with A.J. Banday and K.M. Gorski
(MPA), I have carried out an extensive study in order to examine
whether the source of the reported non-Gaussianity is of cosmological
origin. This study has resulted in plausible resolution to the
problem, tracing the origin of the signal to a systematic effect in
the data. Currently, as an associate of the Planck satellite science
team, I am collaborating with others on developing more sensitive
non-Gaussianity tests suitable for the data this future high
resolution CMB mission will gather.
- Large-Scale Power Spectrum from Peculiar
Velocities: Likelihood analysis
Within the framework of gravitationally induced clustering, velocities
are direct tracers of the total mass density distribution. In
collaboration with A. Dekel, Y. Hoffman (Jerusalem) and I. Zehavi
(Fermilab), I have developed a likelihood technique for measuring the
mass-density power spectrum from galaxy peculiar velocities data
sets. The method has been applied to three galaxy peculiar velocity
catalogs, two consisting mainly of spiral galaxies (Mark-III and SFI)
and the third, in collaboration with L. da Costa (ESO) and his
collaborators, consists of early-type galaxies (ENEAR). All three
yield a value of the rms density fluctuations on the scale of 8 h-1Mpc
of the order of ~1 and mass-density parameter ~Ω0.6 - both higher than
values obtained from other types of data sets, e.g., redshift
surveys. This discrepancy might be attributed to the complicated
nature of galaxy biasing, dominant in redshift catalogs, or to an
inaccuracy in one or more of the analysis method's assumptions.
- Goodness-of-Fit Analysis of Radial Velocities: Principal Component Analysis
In an effort to understand the origin of the aforementioned relatively
high power spectrum amplitude Y. Hoffman (Jerusalem) and I have
performed a Principal Component Analysis and found that the χ2 per
degree of freedom in the uncorrelated eigenspace (defined by the
principal components) is unacceptably low for most of the
eigenmodes. This analysis has called into question our models either
for the theoretical correlation of the radial peculiar velocities, our
understanding of the nature of the measurement errors, or both.
- Reconstruction of the Large Scale Structure
The reconstruction of the linear dynamical fields from various data
sets is a matter of great importance. It enables a reliable comparison
of the density fields obtained from various types of data, such as
redshift galaxy catalogs and peculiar velocity catalogs. Such a
comparison is instrumental in understanding how light traces matter,
and for highlighting interesting areas of activity such as high
density regions, structures obscured by the Galactic plane, etc. In
collaboration with Y. Hoffman (Jerusalem), O. Lahav (IoA Cambridge)
and K. Fisher (IAS, Princeton) I have extended the classical algorithm
of Wiener filtering to perform, in addition to the standard noise
suppression, predictions and dynamical reconstruction of the large
scale structure dynamical fields.
-
Large Scale Structure from CMB,
Redshift and Peculiar velocity Surveys
In the standard cosmological model the primordial perturbation
field is assumed to be homogeneous and isotropic. However, when viewed
in redshift space the line of sight peculiar velocities distort the
galaxy distribution and introduce anisotropy usually termed `redshift
distortion'. Together with Y. Hoffman (Jerusalem), I have calculated a
simple relation between the real and redshift space correlation
functions. This has served as a probe of the density parameter Ω and
the bias parameter.
O. Lahav, D. Lynden-Bell (Cambridge), K. Fisher
(Princeton), Y. Hoffman (Jerusalem), and I have used such a
calculation in order to develop a general reconstruction method of the
primordial perturbation from galaxy redshift surveys.
Together with Y. Hoffman and A. Dekel (Jerusalem) I have applied
Wiener filtering and a constrained realizations algorithm to the
Mark-III peculiar velocities catalog. The method is used to perform
high resolution reconstruction of the density and velocity
fields. Analysis of the tidal velocity field obtained from the
reconstruction reveals a very large bulk flow towards the Shapely
concentration. Currently, I am applying this algorithm to other
velocity data sets. Together with J. Silk (Oxford) and others, we have
pioneered the application of Wiener filtering to CMB data sets, a
method that has since become one of the standard tools in the field.
- The Question of Biasing: Unbiased
Minimal Variance Estimator
The accumulating large redshift surveys are the basic tool for
quantifying the distribution of luminous matter. However, the galaxy
distribution may be biased relative to the underlying mass
distribution. In contrast, the peculiar velocities of galaxies are
presumably honest tracers of the motion of the dark matter. Just as
the rotation curves of galaxies tell us what the density profiles of
the galaxy dark matter halos are, the large scale peculiar velocities
tell us about the density fluctuations of the dark matter in between
galaxies. Given a galaxy formation's recipe (i.e., biasing) and an
assumed value for the matter density parameter, Ω, gravitational
instability relates the two types of data, and permits an assessment
of their mutual consistency. This offers the promise of constraining
Ω and the biasing relation and, more ambitiously, of answering
fundamental questions in cosmology. The simplest biasing scheme is to
assume that the distribution of galaxies linearly follows the
distribution of matter with a constant factor b, called biasing
constant, connecting between them.
One of the major unsettled issues in the study of the large scale
distribution of the luminous matter relative to the mass distribution,
is the inconsistency between the value of the &beta
(= Ω0.6/b) parameter, which reflects the ratio of the
distributions. The value of &beta inferred from comparing the density map,
as constructed from galaxy redshift catalogs, with density map
reconstructed from peculiar velocity catalogs is about 1; while the
value of &beta estimated from a comparison of the radial velocities
obtained from redshift catalogs and from peculiar velocity catalogs is
about 0.5.
In order to settle this issue, I have recently devised a new
statistical reconstruction technique, called 'Unbiased Minimal
Variance Estimation', that recovers the density and the 3D peculiar
velocity fields given a radial peculiar velocity or redshift galaxy
catalogs. Together with E. Branchini (Rome), L.N. da Costa (ESO) and
Y. Hoffman (Jerusalem), I have applied this technique to reconstruct
the smoothed density and peculiar velocity fields from the SEcat
galaxy peculiar velocity catalog and compared them to the density and
3D peculiar velocity field reconstructed from the PSCz galaxy redshift
catalog. These comparisons yield for the first time a consistent
result for the value of the &beta parameter (&beta~0.55).
- Cosmological Parameters from Cluster
Images: Back Projection
A major source of uncertainty in determining the expansion rate of the
Universe, set by the so called Hubble constant, from comparing various
waveband mappings of rich galaxy clusters arises from the asphericity
of the 3-dimensional structure of the density and temperature
distributions of the hot gas. Together with G. Squires (Caltech),
J. Silk (Oxford) and Y. Hoffman (Jerusalem), I have developed a new
method of deprojecting 2-dimensional images assuming axial symmetry in
the 3-dimensional structure. The cluster structures, as derived from
the x-ray, Sunyaev-Zeldovich and gravitational lensing maps, are
combined to constrain the inclination angle of elongated cluster with
respect to the line-of-sight. In principle, for noise-free data, the
gas temperature and density can be determined (non-parametrically) and
compared with the deprojected matter distribution.
It is crucial to
investigate the applicability of the deprojection algorithm for real
cluster images. Together with G. Evrard (Michigan) I have performed a
study of the inversion algorithm on a set of realistic, high
resolution, cluster simulations. The goal is to test the axisymmetric
deprojection algorithm, using as input a representative realization of
the cluster population. The method has performed convincingly on the
simulated cluster images and we expect to soon start applying the
method to real data.
- Temprature of the Universe & the
Lyman-α Forest: Wavelet Analysis
The physical picture emerging from high resolution hydrodynamic
simulations is that the Lyman-a forest is produced mainly by gas with
an overdensity of the order 10. The competition between
photoionization heating and adiabatic cooling produces a very tight
relation between the gas overdensity and temperature. This tight
relation might be used as a very sensitive probe of the intergalactic
medium temperature up to a redshift ~5. In particular, the UV photons
emitted from high redshift quasars may have reionised the
high-redshift Universe.
Together with T. Theuns (IoA Cambridge), I have developed a novel
multi-resolution (wavelet) method for analyzing the thermal evolution
of the intergalactic medium from the observed Lyman-a forest. The
method is designed to characterize he distribution of the Lyman-a line
widths along the spectrum. These widths can be simply related to the
intergalactic medium temperature. The method has been tested on
simulated spectra and proven to work well.
An application of the method to observed spectra convincingly shows a
considerable evolution in the temperature of the intergalactic medium
as a function of redshift. In addition, we have strong evidence for
the occurrence of a substantial increase (about 60%) in the
temperature of the IGM at z~[3-3.5] that is probably related to the
formation of cosmological He-III regions prior to He-II
reionization. We also use the temperature before this jump in order to
constraint the epoch of H-I reionization. The last part of this work
is carried out in collaboration with T-S Kim (ESO), P. Tzanavaris, R.F. Carswell (Cambridge) and J. Schaye (Princeton).
|